A transition metal and carbon monoxide (CO) ligands combine to form coordination compounds known as metal carbonyls. Because of their special bonding properties, these complexes are important in organometallic chemistry and industrial catalysis. The bonding in metal carbonyls can be explained using molecular orbital theory and are both sigma (σ) and pi (π) interactions.
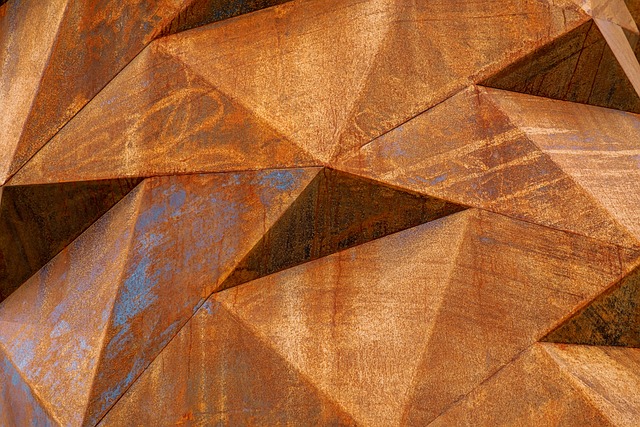
Transition metal carbonyls constitute this class of organometallics. These are homoleptic carbonyns (compounds containing carbonyl ligands only).
Example:
Mononuclear carbonyls:
(i) V(CO)5 – Pentacarbonyvanadium (0)
(ii) Cr(CO)6 – Hexacarbonylchromium (0)
(iii) Mo(CO)6 –Hexacarbonylmolybdenum (0)
(iv) W (CO)6 – Hexacarbonyltungsten (0)
(v) Ni(CO)4 – Tetracarbonylnickel (0)
(vi) Fe(CO)5 – pentacarbonyliron (0).
Polynuclear carbonyls:
(i) Co2(CO)8 – Octacarbonyldicobalt (0)
(ii) Co4(CO)12 – Dodecarbonyltetracobalt (0)
(iii) Mn2(CO)10 – Decarbonyldimaganese (0).
Nature of Bonding in Metal Carbonyls
The synergistic interaction between the metal center and the CO ligands provides the best understanding of the bonding in metal carbonyls. There are two main parts to this interaction:
1.Sigma (σ) Bonding
The carbon monoxide ligand contributes two electrons to the metal’s empty orbital from its lone pair on the carbon atom.
CO is a powerful field ligand because of this donation, which takes place through the carbon end.
This electron donation results in a metal-carbon bond known as a sigma bond.
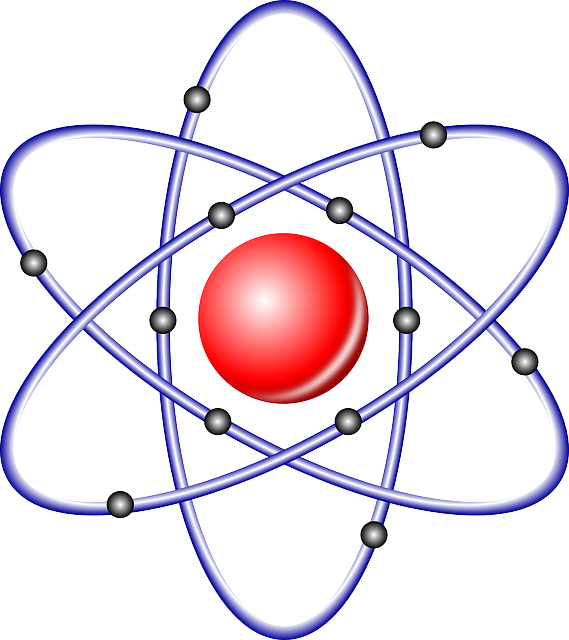
2. Pi (π) Back Bonding
The metal has filled d-orbitals that can interact with the empty anti-bonding π* orbitals of CO, especially at low oxidation states.
The metal-ligand link is strengthened as a result of the back-donation of electron density from the metal to CO.
The carbon-oxygen bond strength decreases as a result of the back-bonding’s reduction of the bond order of CO.
Synergic Effect
The synergic effect is the result of both π-donation from CO to the metal and π-back donation from the metal to CO occurring at the same time. The metal-carbon connection is strengthened while the carbon-oxygen bond is weakened by this dual bonding.
The degree of this effect is determined by a number of variables, including the electronic configuration of the metal, the presence of additional ligands in the coordination sphere, and the metal’s oxidation state (lower oxidation levels promote more back-donation).
Evidence of Bonding in Metal Carbonyls
Several pieces of experimental evidence support the nature of bonding in metal carbonyls:
Infrared (IR) Spectroscopy:
The characteristic stretching frequency of free CO is approximately 2143 cm⁻¹.
Because of π-back donation, this frequency moves lower in metal carbonyl complexes, suggesting a weaker C-O bond.
The frequency of CO stretching decreases as the back donation increases.
Structural Studies:
Metal carbonyls can have a variety of geometries, including octahedral (like Cr(CO)₆), tetrahedral (like Ni(CO)₄), and trigonal bipyramidal (like Fe(CO)₅), according to X-ray crystallography.
The degree of back-bonding affects the bond lengths between metal and carbon.
Types of Metal Carbonyls
Depending on how many metal atoms are present, metal carbonyls can be categorised:
Mononuclear carbonyls, such as Ni(CO)₄, Fe(CO)₅, and Cr(CO)₆, have a single metal center.
• Polynuclear carbonyls, such as Mn₂(CO)₁₀ and Fe₂(CO)₉, have many metal centers and frequently show metal-metal bonding.
Applications of Metal Carbonyls
1.Catalysis: In industrial processes like Fischer-Tropsch synthesis and hydroformylation, metal carbonyls are employed as catalysts.
2. Organometallic Chemistry: These substances are vital building blocks for organometallic processes.
3. CO Gas Transport: Research on the storage and transportation of CO gas makes use of certain metal carbonyls.
Summary
Metal carbonyls are essential in both theoretical and applied chemistry because of the special synergistic interaction that occurs during bonding between metal and CO ligands. Stability and reactivity are essential for industrial and catalytic applications and are defined by the dual nature of sigma donation and pi back-donation.
Note
Synergic effect is the characteristic property of back bonding that stabilizes (or strengthens) the metal ligand interaction.
Stability of coordination compounds : The stability of a complex in solution refers to the degree of association between the two species involved in the state of equilibrium.
M + 4L – ML4
Magnitude of the equilibrium constant quantitatively expresses the stability.
Stability depends on.
(i) Charge on the central metal ion (oxidation state): Greater the charge on central metal ion, more is the stability.
(ii) Basic nature of ligand : More the basic strength of ligand, more is the stability of complex.
(iii) Presence of chelate rings : Formation of chelate ring increases the stability of complex.
(iv) Size of the metal ion : Smaller the size metal ion, more is stability.
(v) Electronegativity and polarizing power of the central metal ion : More is the electronegativity and polarizing power of the metal ion, more stable is the complex.
A coordination compound made up of a transition metal bound to carbon monoxide (CO) ligands is called a metal carbonyl. Ni(CO)₄ and Fe(CO)₅ are two examples.
Both pi (π) back bonding, in which the metal provides electrons to CO’s antibonding orbitals, and sigma (σ) bonding, in which CO donates electrons to the metal, are present in metal carbonyls.
When σ-donation from CO to the metal and π-back donation from the metal to CO occur simultaneously, the metal-carbon bond is strengthened and the carbon-oxygen link is weakened. This phenomenon is known as the synergic effect.
The CO stretching frequency is shifted from its free value (- 2143 cm⁻¹) according to IR spectroscopy. Strong π-back bonding is indicated by a decreased CO stretching frequency in metal carbonyls.
The coordination number and metal hybridization determine the shape of metal carbonyls. A tetrahedral structure is formed by Ni(CO)₄ (sp³ hybridized), whereas a trigonal bipyramidal shape is formed by Fe(CO)₅ (sp³d hybridized).
The metal’s oxidation state (more back-bonding is preferred in a lower oxidation state).
The metal d-orbitals’ electron density.
The characteristics of the additional ligands that make up the complex.
Catalysis (such as the Fischer-Tropsch process or hydroformylation).
Materials used as precursors in organometallic chemistry.
Transport and storage of carbon monoxide gas in research.