When a thermodynamic system transits from one state to another, it goes through a number of changes, which are called as thermodynamic processes. Variables are pressure, volume, temperature, and internal energy are frequently altered during these operations. In physics, engineering, and chemistry, thermodynamic processes are vital because they help us to understand how energy is moved and transformed in physical systems.
Basic Concepts of Thermodynamic Processes
The state of a thermodynamic system is determined by quantifiable characteristics such as temperature, pressure, and volume. A thermodynamic process takes place when one or more of these characteristics alter as a result of the system’s interaction with its environment. Heat transfer, may be a part of this interaction.
Quasi Static process: An idealized process in which at every stage the system is an equilibrium state. Such a process is infinitely slow hence the name quasi-static. The system changes its variable (P,T,V) so slowly that remains in thermal and mechanical equilibrium with its surroundings throughout.
Following are some important special thermodynamic process:
Type of process | Feature |
Isothermal | Temperature constant |
Isobaric | Pressure constant |
Isochoric | Volume constant |
Adiabatic | No heat flow between the system and surroundings (ΔQ = 0) |
Types of Thermodynamic Processes
Four main categories can be used to broadly classify thermodynamic processes, each of which is identified by the circumstances in which it takes place.
1. Isothermal Process (Constant Temperature)
The system keeps its temperature steady during the transition in an isothermal process. The system’s internal energy does not change because the temperature does not change. The technology completely transforms any heat that is introduced or removed into work. The equation that represents the ideal gas law: PV = nRT
Since temperature is constant, the relationship between pressure and volume is described by: P1V1 = P2V2
where P and V denote the pressure and volume at the initial and final states, respectively.
2. Adiabatic Process (No Heat Exchange)
There is no heat transfer between the system and its environment during an adiabatic process. Since the system is thermally insulated, all energy changes are the consequence of work done by or on the system itself. The internal energy change in such a process is equivalent to the labour performed. ΔU = −W
For an ideal gas undergoing an adiabatic process, the relationship between pressure and volume is given by: PVγ = constant
where γ is the heat capacity ratio (specific heat at constant pressure divided by specific heat at constant volume).
3. Isobaric Process (Constant Pressure)
Heat exchange between the system and its surroundings is made possible by an isobaric process that takes place at constant pressure. The pressure does not change during this process, but the volume cgange. The work done by the system in an isobaric process is expressed as: W = PΔV
Where ΔV is the change in volume. Since heat is added or removed, there is a change in the internal energy and the enthalpy of the system.
4. Isochoric Process (Constant Volume) The isochoric process is a process that takes place at the constant volume (V = Constant, dV = 0) When a process is isochoric, the system’s volume stays constant, indicating that the system does not perform any work.
W = 0
Any heat added to or removed from the system results solely in a change in its internal energy: Q = ΔU
Since volume is fixed, changes in pressure and temperature occur as the system absorbs or releases heat.
Reversible and Irreversible Processes
A thermodynamic process can also be classified as reversible or irreversible:
Reversible Processes: The system can always remain in equilibrium since these processes proceed endlessly slowly. Although they are theoretical benchmarks, these ideal procedures are unachievable in practical settings.
• Irreversible Processes: These processes, which include dissipative effects like heat loss, turbulence, and friction, happen on their own. Although they happen in natural systems and are more realistic, they are not as effective as reversible processes.
Applications of Thermodynamic Processes
Heat engines use cyclic thermodynamic processes to convert heat into work; refrigeration systems use adiabatic and isothermal processes to transfer heat from lower to higher temperatures; and power plants use thermodynamic cycles to generate electricity by transforming thermal energy into mechanical work.
Note
Polytropic process
Polytropic process follows the equations of the form PVx = constant where x is a constant called polytropic index of compression or expansion x can any have value between
–α to + α.
The equation PVx = constant is analogous to the equation PVγ = constant for adiabatic process.
Adiabatic process is a special case of polytropic process with x = γ.
The equation for the polytropic process are
(i) PVx = constant
(ii) TVx-1 = constant
(iii) p1-x Tx = constant
For x = 0 process is isobaric (P = constant)
For x = 1 process is isothermal (PV = constant)
For x = γ process is adiabatic (PVγ = constant).
Conclusion
Energy is converted and used in different systems requires a thermodynamic processes. These processes determine the behaviour and effectiveness of thermodynamic systems, whether they are used to power an automobile engine, produce electricity, or regulate the temperature in a refrigerator.
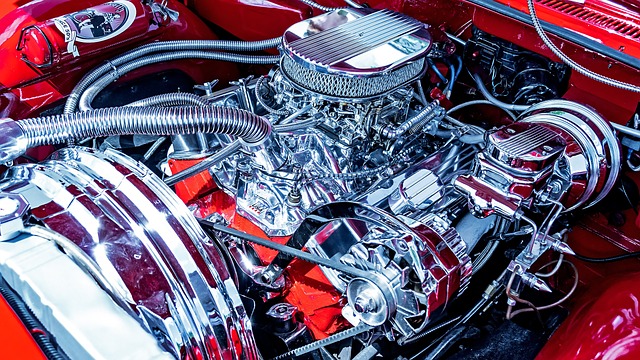
When a system moves from one equilibrium state to another, it goes through a series of changes known as a thermodynamic process. Heat transfer, work, or both may cause changes in the system’s pressure, volume, temperature, and internal energy during this process.
The four primary types of thermodynamic processes are:
Isothermal Process: Occurs at constant temperature.
Adiabatic Process: No heat exchange with the surroundings.
Isobaric Process: Takes place at constant pressure.
Isochoric Process: Volume remains constant, with no work done.
Reversible Process: Always maintains equilibrium while proceeding indefinitely slowly. Although it is ideal and extremely effective, it is not feasible to accomplish in real life.
• Irreversible Process: More realistic but less effective than a reversible process, this process occurs spontaneously and has dissipative effects like friction and heat loss.