Valence Shell Electron Pair Repulsion (VSEPR) theory that helps to predict the shape of molecules based on the arrangement of electron pairs around a central atom. Developed by Ronald Gillespie and Ronald Nyholm in 1957, it is widely used to explain molecular geometry and bonding in both simple and complex molecules.
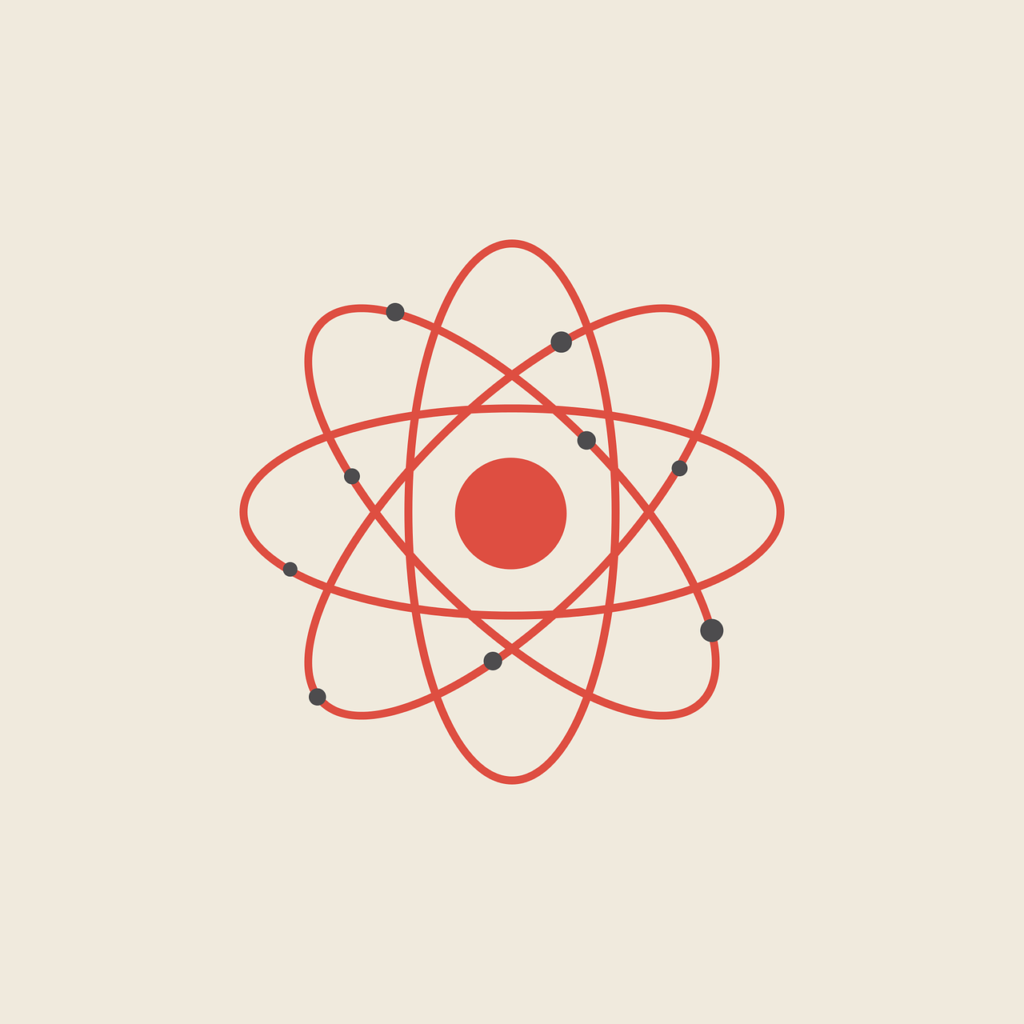
What is the Valence Shell Electron Pair Repulsion (VSEPR) Theory?
The VSEPR theory is based on the idea that electron pairs around a central atom will repel each other. These pairs of electrons, whether involved in bonds or as lone pairs (non-bonding pairs), tend to arrange themselves in a way that minimises repulsion. This arrangement determines the shape of the molecule.
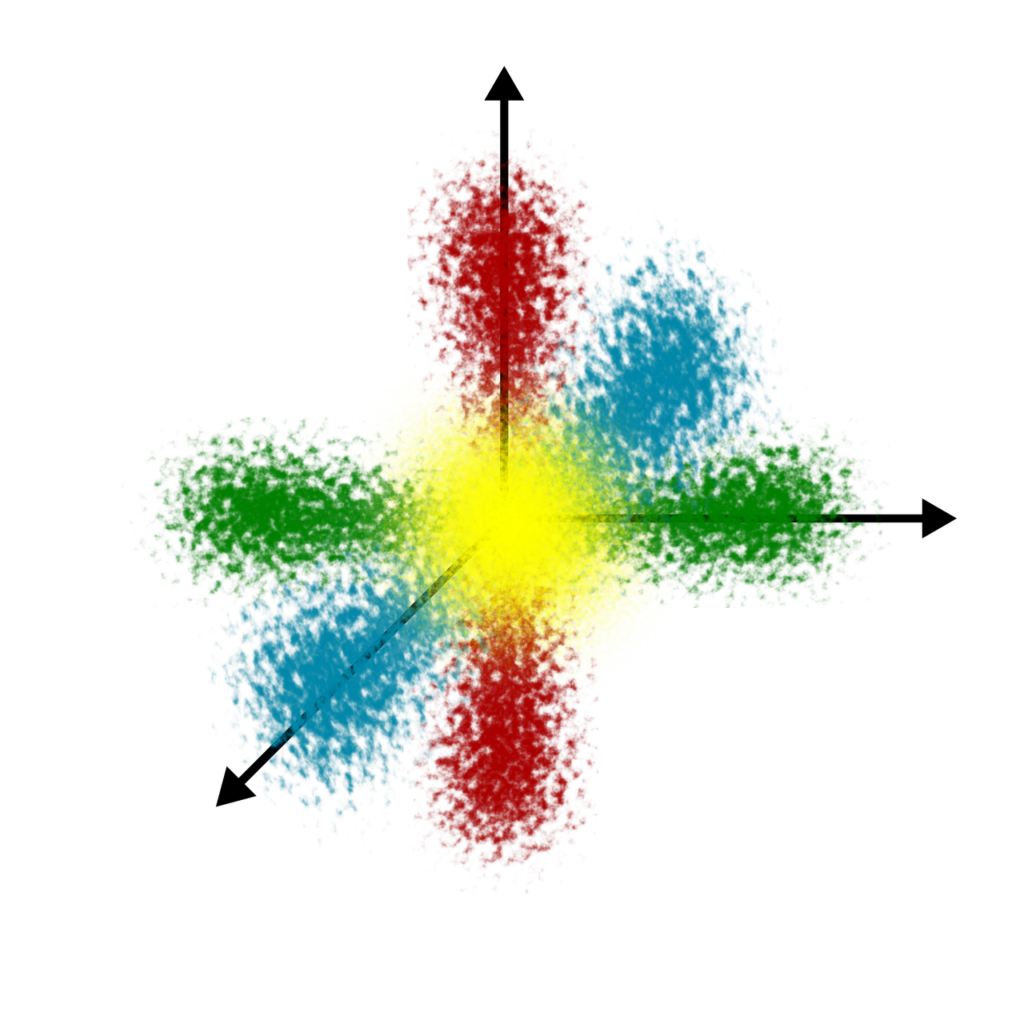
Principle is simple: Like charges repel each other. As electrons are negatively charged, they push away from one another as far apart as possible, giving the molecule a specific 3D shape.
Key assumption of the VSEPR Theory
1.Electron Pair Repulsion: Both bonding and lone pairs of electrons around the central atom repel one another.
2.Minimising Repulsion: Molecule adopts a geometry that places the electron pairs as far as possible to minimise repulsion.
3. Effect of Lone Pairs: Lone pairs occupy more space than bonding pairs because they are not shared atoms, which can distort the geometry of the molecule.
4. Multiple Bonds: Double or triple bonds are treated as a single region of electron density.
Types of Electron Pairs
1.Bonding Pairs: These are shared electrons that form bonds between atoms.
2.Lone Pairs: These are unshared electrons localized on a single atom.
The geometry of a molecule depends on the total number of regions of electron density (bonding and lone pairs) around the central atom.
Common Molecular Geometries
1.Linear (180°): Molecules with two regions of electron density (e.g., CO₂).
2. Trigonal Planar (120°): Molecules with three regions of electron density (e.g., BF₃).
3. Tetrahedral (109.5°): Molecules with four regions of electron density (e.g., CH₄).
4. Trigonal Bipyramidal (120°, 90°): Molecules with five regions of electron density (e.g., PCl₅).
5. Octahedral (90°): Molecules with six regions of electron density (e.g., SF₆).
Influence of Lone Pairs
Lone pairs repel more strongly than bonding pairs, causing distortions in ideal geometries. For example:
Ammonia (NH₃): Though the electron geometry is tetrahedral, the presence of one lone pair reduces the bond angles slightly, resulting in a trigonal pyramidal shape.
Water (H₂O): With two lone pairs, the bond angles shrink and giving a bent or angular shape.
Applications
1.Methane (CH₄): With four bonding pairs and no lone pairs, methane has a tetrahedral geometry.
2.Carbon Dioxide (CO₂): With two double bonds and no lone pairs, CO₂ adopts a linear geometry.
3.Sulfur Hexafluoride (SF₆): With six bonding pairs, SF₆ takes on an octahedral geometry
Limitations of Valence Shell Electron Pair Repulsion (VSEPR) Theory
VSEPR theory is excellent for predicting molecular shapes, it has some limitations:
1.No Quantitative Predictions: It cannot calculate bond angles precisely.
2. No Bond Strength Information: It doesn’t deal with bond strength or energy.
3.Exceptions: Complex molecules with d-orbitals or unusual electronic distributions may not follow VSEPR predictions accurately.
Why is Valence Shell Electron Pair Repulsion (VSEPR) Theory?
Molecular shape is vital in fields like biochemistry, pharmacology, and materials science. Molecular geometry influences properties like polarity, reactivity, and intermolecular forces:
Bent shape of water molecules explains its polarity and excellent solvent properties.
Linear geometry of CO₂ makes it nonpolar, despite having polar bonds.
Note:-
Valence Shell Electron Pair Repulsion (VSEPR) Theory is a straightforward and a powerful tool for understanding molecular shapes.
Electron pair repulsions, it allows chemists to visualise and predict the 3D arrangement of atoms in a molecule.
Shape of molecule depend upon the number of valence shell electron pairs around the central atom.
Electrons pairs in the valence shell repel one another.
Electrons pairs tends to occupy such positions in space that minimise repulsion and maximise distance between them.
Where two or more resonance structures an represent a molecule, the VSEPR model is applicable to any such structure
The VSEPR theory (Valence Shell Electron Pair Repulsion theory) predicts the shapes of molecules by assuming that electron pairs around a central atom repel each other. This repulsion forces the electron pairs to arrange themselves as far apart as possible, minimizing repulsion and determining the molecule’s geometry.
Electron pairs, both bonding and non-bonding (lone pairs), repel each other.
Molecules adopt a geometry that minimizes these repulsions.
Lone pairs exert greater repulsive forces than bonding pairs.
Multiple bonds are treated as a single region of electron density.
Lone pairs occupy more space than bonding pairs because they are localised on one atom. This stronger repulsion can distort bond angles, making the geometry deviate from ideal shapes. For example, in water (H₂O), the two lone pairs reduce the bond angle, resulting in a bent geometry.
Linear: 180° bond angle (e.g., CO₂)
Trigonal Planar: 120° bond angle (e.g., BF₃)
Tetrahedral: 109.5° bond angle (e.g., CH₄)
Trigonal Pyramidal: ~107° bond angle (e.g., NH₃)
Bent/Angular: <120° or <109.5° bond angles (e.g., H₂O)
VSEPR theory treats multiple bonds (double or triple bonds) as a single region of electron density. For example, the double bonds in carbon dioxide (CO₂) count as one region each, resulting in a linear shape.
It cannot predict precise bond angles or account for bond strength.
It may not accurately predict the shapes of complex molecules involving transition metals or d-orbitals.
It does not consider the energy or hybridization of bonds.